Imagine if the brain power of the device you’re reading this on was so advanced that it could store information as multiple things simultaneously?
As you might’ve guessed, we’re back in the weird and wonderful world of quantum physics, here to examine a whole new platform in quantum information via quDits and qubits.
In a recently published paper in Nature, University of Varennes researcher Michael Kues and colleagues demonstrated how photons can become potent quantum resources when produced on a photonic chip in the shape of colour-entangled quDits.
If you think of regular qubits as being black and white, quDits would be their cousins that can exist in any colour of the rainbow. The results could have profound implications for quantum computing, given the advance in processing power and unprecedented scale of calculations.
This is all typical quantum gobbledygook, indeed. Let’s unpack it.
What are qubits?
You may have heard the term qubit before. It stands for a quantum bit, and it’s the most basic unit of information on a quantum computer. To understand how quDits work, we first need to understand qubits.
The device you’re reading this on performs processes using classical bits, that being zero or one, and they follow a linear pattern. Very much a black and white world.
Quantum computers, on the other hand, delve into the realm of subatomic particles and their quantum states to store information.
Qubits can be zero or one at the same time, lending far superior power to these machines since their capacity for extremely complex calculations is far greater. Photons, electrons, ions and nuclei can all be used as qubits.
As an example of integration, consider the electron. Electrons have magnetic fields, so they are like tiny little magnets themselves, and this property is known as ‘spin’.
So if you place one inside a magnetic field, it will align with that field no different to how a compass would with the magnetic field of Earth.
This is the lowest energy state for an electron, and is aptly called ‘spin down’. Its value is theoretically zero, or else the tiniest bit above that according to interference from energy.
Forcing it into its theoretically highest energy state, an ‘up spin’, takes energy. Think of it like twisting the dial on your compass to point north. Either way, its state is defined as one or the other.
The quantum superposition
So far, this is the same as the two separate states of one and zero on a standard computer. But as we know, in the quantum world, things can exist simultaneously, so the spin could be up or down before we measure it.
Therein we have the quantum superposition; it could be in any multiple of states at any time in this sphere of possibilities. Why? Because it has a value of both one and zero, and any value between them.
Abstractly speaking, it might seem that a qubit contains an infinite amount of information because its value can encode an infinite sequence of digits.
With zero and one as the parameters, there are infinite possibilities when decimal points come on board. An in-between number could be as simple as 0.9, or as crazy as 0.64926509473949693962.
Quantum mechanics has an important rule, however. Even though the value of a qubit can float in this limbo of possible values, a measurement, once extracted, must be an ordinary bit – a classical one or zero.
The implications for computing are enormous. In a game of chess, a quantum computer would be able to analyze all possibilities at once and simply follow through with the best option. A standard computer can only analyze situations step by step.
Quantum devices need to be kept at unbelievably cold temperatures so that their atoms don’t get knocked about by electromagnetic waves – they’re stored at absolute zero (-273℃). Not only that, but cell phones aren’t allowed anywhere near because their microwaves could cause the qubit superposition to collapse.
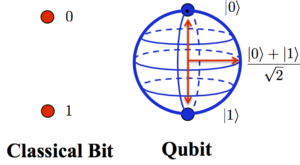
Quantum amigos
When qubits make friends with other qubits, they become entangled, and this is where things start to get exponential and crazy.
If two are entangled, they share a similar value, the possibilities begin to multiply, and every qubit that gets thrown into the mix just doubles the processing capabilities. Two entangled qubits can make four calculations simultaneously; three can make eight calculations and so on.
Now here’s a mind-blowing fact: If you had 300 qubits, i.e. 2300, you could conduct more parallel computations than there are atoms in the known universe.
This is all far too complex and abstract, so let’s discuss the applications.
- Encryption: We can’t directly measure or observe qubits, so therein lies the opportunity for quantum cryptography. The unfathomable amount of possibilities could revolutionize data security. Or on the flip side, you could use quantum computers to break those very encryptions.
- Simulations: The interactions between molecules and atoms can be studied at an unprecedented level of detail using this technology. This allows us to develop better drugs and materials.
- Medicine: Quantum computers analyze monumental amounts of data and possibilities at once, so we can tackle complex problems like mapping out proteins in their entirety. This is invaluable for designing treatments for diseases and ailments like cancer.
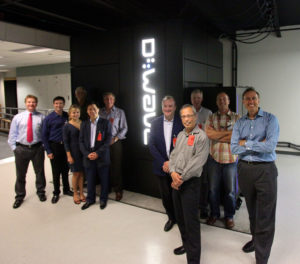
Enter the mighty quDit
Now researchers have created a microchip with two entangled quDits, both in possession of 10 states, totalling 100 dimensions. QuDits are the measuring unit of quantum information in a d-level system; that is, a system where the number of states is greater than two (expressed on paper as D>2).
Qudits are composed using photons exclusively, and for good reason. Photons are both a particle and a wave at the same time – a phenomenon known as the particle-wave duality.
An entangled, high-dimensional photon could be blue, yellow, green, or red, all at the same time. The amount of information that can be stored on a single photon is therefore exponentially greater.
While qubit superpositions are incredibly fragile, the team managed to send entangled photons through a 24-km-long optical fibre telecommunications system without the photons losing their coherence. Good luck trying to hack quantum communications applications on that scale.
To open this web page, your computer used classical bits while communicating with a server potentially thousands of miles away. When a telecommunications system capable of maintaining the coherence of quDits is developed, this will have enormous implications for computing, both regular and mind-bending.
“While this will take some additional years, it is the final step required to achieve systems that can outperform classical computers and communications,” says Kues.